Origin of life
- This article focuses on modern scientific research on the origin of life on Earth, rather than religious belief, creation myths, or the specific concept of spontaneous generation.
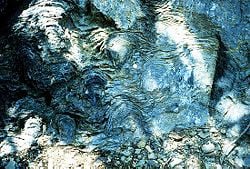
The origin of life, in the physical, chemical, and biological sciences, is the process by which life originated on Earth from non-living matter. It addresses questions of how, when, and what, and includes a variety of hypotheses regarding this development.
Abiogenesis (Greek a-bio-genesis, "non biological origins") is, in its most general sense, the generation of life from non-living matter, and includes both the archaic concept of spontaneous generation and the modern concepts of origination of life on Earth. Classical notions of abiogenesis, now known as spontaneous generation, held that complex, living organisms are generated by decaying organic substances, for example, that mice spontaneously appear in stored grain or maggots spontaneously appear in meat. Today, abiogenesis is primarily used to refer to hypotheses about the chemical origin of life, such as from a primordial sea or in the vicinity of hydrothermal vents, and most probably through a number of intermediate steps, such as non-living but self-replicating molecules (biopoiesis).
The topic "origin of life" also encompasses possible extra-planetary or extra-terrestrial origin of life hypotheses.
This article will focus on modern scientific research on the origin of life. In this respect, the various models ignore religious or spiritual beliefs, such as creation by a Supreme Being, whether working through natural law or supernatural agency. Lee (1981), for example, advances the notion that the physical process from abiotic minerals to life is the external manifestation of a process guided by internal spiritual forces.
The various scientific models are necessarily speculative. Proposals for the origin of life remain at the stage of hypotheses, meaning they are working assumptions for scientists researching how life began. If test results provide sufficient support for acceptance of a hypothesis, then that is the point at which it would become a theory.
Origin of life studies is a limited field of research, despite its profound impact on biology and human understanding of the natural world. Progress in this field is generally slow and sporadic, though it still draws the attention of many due to the eminence of the question being investigated. A few facts give insight into the conditions in which life may have emerged, but the mechanisms by which non-life became life are still elusive.
Spontaneous generation
A cornerstone principle of biology is that living organisms come only from other living organisms (excepting the original appearance of life on Earth). However, historically people relying on their observations came to the conclusion that living organisms could arise virtually overnight from rotting meat (flies), stored grain (mice), mud (fish), clear broth (bacteria), and so forth. This provided one explanation for the origin of life on earth, albeit identifying the manner rather than the mechanics.
According to Aristotle, it was a readily observable truth that aphids arise from the dew that falls on plants, fleas from putrid matter, mice from dirty hay, and so forth. In the seventeenth century, such assumptions started to be questioned, such as by Sir Thomas Browne in his Pseudodoxia Epidemica of 1646. His conclusions were not widely accepted; for example, his contemporary, Alexander Ross, wrote: "To question this (i.e., spontaneous generation) is to question reason, sense, and experience. If he doubts of this, let him go to Egypt, and there he will find the fields swarming with mice, begot of the mud of Nylus, to the great calamity of the inhabitants."
However, experimental scientists continued to decrease the conditions within which the spontaneous generation of complex organisms could be observed. These include the work of Francesco Redi, who in 1668, proved that no maggots appeared in meat when flies were prevented from laying eggs; Lazzaro Spallanzani, who in 1768, showed that microorganisms could not appear in flasks of boiled broth left sealed; and Louis Pasteur's experiments in 1862, which disproved that organisms such as bacteria and fungi appear in nutrient rich media of their own accord. With the work of Pasteur, the doctrine of spontaneous generation, in the sense of a continuing process of generation of life from non-living matter, "died a sudden death" (Towle 1989).
However, the obvious conclusion is that at some point, life on Earth must have originated, and thus some form of "spontaneous generation" must have occurred.
History of the concept of the origin of life in science
In a letter to Joseph Dalton Hooker of February 1 1871, Charles Darwin made the suggestion that the original spark of life may have begun in a "warm little pond, with all sorts of ammonia and phosphoric salts, lights, heat, electricity, etc. present, [so] that a protein compound was chemically formed ready to undergo still more complex changes." He went on to explain that "at the present day such matter would be instantly devoured or absorbed, which would not have been the case before living creatures were formed." In other words, the presence of life itself prevents the spontaneous generation of simple organic compounds from occurring on Earth today—a circumstance that makes the search for the origin of life dependent on the sterile conditions of the laboratory.
An experimental approach to the question was beyond the scope of laboratory science in Darwin's day, and no real progress was made until 1923, when Aleksandr Ivanovich Oparin surmised that it was the presence of atmospheric oxygen and other more sophisticated life-forms that prevented the chain of events that would lead to the appearance of life. In his The Origin of Life on Earth, Oparin suggested that the primitive Earth contained an atmosphere of hydrogen gas, water vapor, ammonia, and organic molecules of carbon and hydrogen, such as methane (Towle 1989). He argued that a "primeval soup" of organic molecules, such as amino acids, could be created in an oxygen-less atmosphere through the action of sunlight, at temperatures above the boiling point of water. These organic molecules would combine in ever-more complex fashion until they dissolved into a coacervate droplet, collecting in the lakes and seas formed when the Earth cooled and lakes and seas formed. These droplets would "grow" by fusion with other droplets, and "reproduce" through fission into daughter droplets, and so have a primitive metabolism in which those factors which promote "cell integrity" survive, those that do not become extinct.
All modern theories of the origin of life take Oparin's ideas as a starting point.
Current models
There is no truly "standard" model of the origin of life. But most currently accepted models build in one way or another upon a number of discoveries about the origin of molecular and cellular components for life, which are listed in a rough order of postulated emergence:
- Plausible pre-biotic conditions result in the creation of certain basic small molecules (monomers) of life, such as amino acids. This was demonstrated in the Miller-Urey experiment by Stanley L. Miller and Harold C. Urey in 1953, although it is now generally held that their laboratory conditions did not reflect the original Earth's atmosphere.
- Phospholipids (of an appropriate length) can spontaneously form lipid bilayers, a basic component of the cell membrane.
- The polymerization of nucleotides into random RNA molecules might have resulted in self-replicating ribozymes (RNA world hypothesis).
- Selection pressures for catalytic efficiency and diversity result in ribozymes, which catalyse peptidyl transfer (hence formation of small proteins), since oligopeptides complex with RNA to form better catalysts. Thus the first ribosome is born, and protein synthesis becomes more prevalent.
- Protein out-compete ribozymes in catalytic ability, and therefore become the dominant biopolymer. Nucleic acids are restricted to predominantly genomic use.
There are many different hypotheses regarding the path that might have been taken from simple organic molecules to protocells and metabolism. Many models fall into the "genes-first" category or the "metabolism-first" category, but a recent trend is the emergence of hybrid models.
The origin of the basic biomolecules, while not settled, is less controversial than the significance and order of steps 2 and 3. The basic chemicals from which life was thought to have formed are commonly held to be methane (CH4), ammonia (NH3), water (H2O), hydrogen sulfide (H2S), carbon dioxide (CO2) or carbon monoxide (CO), and phosphate (PO43-). Molecular oxygen (O2) and ozone (O3) typically are considered to have been either rare or absent.
As of 2007, no one had yet synthesized a "protocell" using basic components that would have the necessary properties of life (the so-called "bottom-up-approach"). Without such a proof-of-principle, explanations have tended to be short on specifics. However, some researchers working in this field have argued that a "top-down approach" is more feasible. One such approach involves engineering existing prokaryotic cells with progressively fewer genes, attempting to discern at which point the most minimal requirements for life were reached. The biologist John Desmond Bernal coined the term biopoesis for this process, and suggested that there were a number of clearly defined "stages" that could be recognized in explaining the origin of life.
Stage 1: The origin of biological monomers
Stage 2: The origin of biological polymers
Stage 3: The evolution from molecules to cell
Bernal suggested that Darwinian evolution may have commenced early, some time between Stage 1 and 2.
Origin of organic molecules
Miller's experiments
In 1953, a graduate student, Stanley Miller, and his professor, Nobel Prize-winning chemist Harold Urey, performed an experiment that was taken as support for the view that organic molecules could have spontaneously formed on early Earth from inorganic precursors. The now-famous "Miller-Urey experiment" used a highly reduced (as opposed to oxidizing) mixture of gases—methane, ammonia, and hydrogen—to form basic organic monomers. Initially recovering such amino acids as glycine and alanine, two of the simplest amino acids found in proteins, he subsequently repeated the experiment and he and others obtained small amounts of many biologically important amino acids, including additional organic compounds (Wells 2000). Whether the mixture of gases used in the Miller-Urey experiment truly reflects the atmospheric content of early Earth is a controversial topic. Other less reducing gases produce a lower yield and variety.
Wells (2000), for example, notes that "most geochemists have been convinced that the experiment failed to simulate conditions on the early Earth," and he presents several geochemical and biochemical studies and theoretical models that infer appreciable amounts of molecular oxygen, and that the primitive methane-ammonia atmosphere did not exist. The presence of appreciable amounts of oxygen in the prebiotic atmosphere would have essentially prevented the formation of organic molecules. However, others conclude there was not appreciable amounts of oxygen.
In 1983, Miller reported that he could produce the simplest amino acid, glycine, in an atmosphere containing carbon monoxide and carbon dioxide instead of methane, as long as free hydrogen was present, but that was the best they could do in the absence of methane (Wells 2000).
In 2006, another experiment suggested that a thick, organic haze might have blanketed early Earth. An organic haze can form over a wide range of methane and carbon dioxide concentrations, believed by many to be present in the atmosphere of primitive Earth. After forming, these organic molecules presumably would have floated down all over the Earth, allowing life to flourish globally.
Simple organic molecules are, of course, a long way from a fully functional, self-replicating life form. But in an environment with no preexisting life, these molecules may have accumulated and provided a rich environment for chemical evolution ("primeval soup theory"). On the other hand, the spontaneous formation of complex polymers from abiotically generated monomers under these conditions is not at all a straightforward process. Besides the necessary basic organic monomers, also compounds that would have prohibited the formation of polymers were formed in high concentration during the experiments.
Other sources of complex molecules have been postulated, including sources of extra-terrestrial stellar or interstellar origin. For example, from spectral analyses, organic molecules are known to be present in comets and meteorites. In 2004, a team detected traces of polycyclic aromatic hydrocarbons (PAH's) in a nebula, the most complex molecule, to that date, found in space. The use of PAH's has also been proposed as a precursor to the RNA world in the PAH world hypothesis.
It can be argued that the most crucial challenge unanswered by this theory is how the relatively simple organic building blocks polymerise and form more complex structures, interacting in consistent ways to form a protocell. For example, in an aqueous environment, hydrolysis of oligomers/polymers into their constituent monomers would be favored over the condensation of individual monomers into polymers. Also, the Miller experiment produced many substances that would undergo cross-reactions with the amino acids or terminate the peptide chain.
Eigen's hypothesis
In the early 1970s, a major attack on the problem of the origin of life was organized by a team of scientists gathered around Manfred Eigen of the Max Planck Institute. They tried to examine the transient stages between the molecular chaos in a prebiotic soup and the transient stages of a self replicating hypercycle, between the molecular chaos in a prebiotic soup and simple macromolecular self-reproducing systems.
In a hypercycle, the information storing system (possibly RNA) produces an enzyme, which catalyzes the formation of another information system, in sequence until the product of the last aids in the formation of the first information system. Mathematically treated, hypercycles could create quasispecies, which through natural selection entered into a form of Darwinian evolution. A boost to hypercycle theory was the discovery that RNA, in certain circumstances forms itself into ribozymes, a form of RNA enzyme.
Wächtershäuser's hypothesis
Another suggested answer to this polymerization conundrum was provided in 1980s, by Günter Wächtershäuser in his iron-sulfur world theory. In this theory, he postulated the evolution of (bio)chemical pathways as fundamentals of the evolution of life. Moreover, he presented a consistent system of tracing today's biochemistry back to ancestral reactions that provide alternative pathways to the synthesis of organic building blocks from simple gaseous compounds.
In contrast to the classical Miller experiments, which depend on external sources of energy (such as simulated lightning or UV irradiation), "Wächtershäuser systems" come with a built-in source of energy, sulfides of iron, and other minerals (such as pyrite). The energy released from redox reactions of these metal sulfides is not only available for the synthesis of organic molecules, but also for the formation of oligomers and polymers. It is therefore hypothesized that such systems may be able to evolve into autocatalytic sets of self-replicating, metabolically active entities that would predate the life forms known today.
The experiment, as performed, produced a relatively small yield of dipeptides (0.4 to 12.4 percent) and a smaller yield of tripeptides (0.003 percent) and the authors note that: "Under these same conditions dipeptides hydrolysed rapidly." Another criticism of the result is that the experiment did not include any organomolecules that would most likely cross-react or chain-terminate (Huber and Wächtershäuser 1998).
The latest modification of the iron-sulfur-hypothesis was provided by William Martin and Michael Russell in 2002. According to their scenario, the first cellular life forms may have evolved inside so-called black smokers at seafloor spreading zones, in the deep sea. These structures consist of microscale caverns that are coated by thin membraneous metal sulfide walls. Therefore, these structures would solve several critical points of the "pure" Wächtershäuser systems at once:
- The micro-caverns provide a means of concentrating newly synthesized molecules, thereby increasing the chance of forming oligomers;
- The steep temperature gradients inside a black smoker allow for establishing "optimum zones" of partial reactions in different regions of the black smoker (e.g. monomer synthesis in the hotter, oligomerization in the colder parts);
- The flow of hydrothermal water through the structure provides a constant source of building blocks and energy (freshly precipitated metal sulfides);
- The model allows for a succession of different steps of cellular evolution (prebiotic chemistry, monomer and oligomer synthesis, peptide and protein synthesis, RNA world, ribonucleoprotein assembly and DNA world) in a single structure, facilitating exchange between all developmental stages;
- Synthesis of lipids as a means of "closing" the cells against the environment is not necessary, until basically all cellular functions are developed.
This model locates the "last universal common ancestor" (LUCA) inside a black smoker, rather than assuming the existence of a free-living form of LUCA. The last evolutionary step would be the synthesis of a lipid membrane that finally allows the organisms to leave the microcavern system of the black smokers and start their independent lives. This postulated late acquisition of lipids is consistent with the presence of completely different types of membrane lipids in archaebacteria and eubacteria (plus eukaryotes) with highly similar cellular physiology of all life forms in most other aspects.
Another unsolved issue in chemical evolution is the origin of homochirality; that is, all monomers having the same "handedness" (amino acids being left handed, and nucleic acid sugars being right handed). Homochirality is essential for the formation of functional ribozymes (and probably proteins too). The origin of homochirality might simply be explained by an initial asymmetry by chance, followed by common descent. Work performed in 2003, by scientists at Purdue identified the amino acid serine as being a probable root cause of organic molecules' homochirality. Serine forms particularly strong bonds with amino acids of the same chirality, resulting in a cluster of eight molecules that must be all right-handed or left-handed. This property stands in contrast with other amino acids, which are able to form weak bonds with amino acids of opposite chirality. Although the mystery of why left-handed serine became dominant is still unsolved, this result suggests an answer to the question of chiral transmission: how organic molecules of one chirality maintain dominance once asymmetry is established.
From organic molecules to protocells
The question, "How do simple organic molecules form a protocell?" is largely unanswered, but there are many hypotheses. Some of these postulate the early appearance of nucleic acids ("genes-first") whereas others postulate the evolution of biochemical reactions and pathways first ("metabolism-first"). Recently, trends are emerging to create hybrid models that combine aspects of both.
"Genes first" models: The RNA world
The RNA world hypothesis suggests that relatively short RNA molecules could have spontaneously formed that were capable of catalyzing their own continuing replication.
It is difficult to gauge the probability of this formation. A number of theories of modes of formation have been put forward. Early cell membranes could have formed spontaneously from proteinoids, protein-like molecules that are produced when amino acid solutions are heated—when present at the correct concentration in aqueous solution, these form microspheres that are observed to behave similarly to membrane-enclosed compartments. Other possibilities include systems of chemical reactions taking place within clay substrates or on the surface of pyrite rocks.
Factors supportive of an important role for RNA in early life include its ability to replicate; its ability to act both to store information and catalyze chemical reactions (as a ribozyme); its many important roles as an intermediate in the expression and maintenance of the genetic information (in the form of DNA) in modern organisms; and the ease of chemical synthesis of at least the components of the molecule under conditions approximating the early Earth.
A number of problems with the RNA world hypothesis remain, particularly the instability of RNA when exposed to ultraviolet light, the difficulty of activating and ligating nucleotides, the lack of available phosphate in solution required to constitute the backbone, and the instability of the base cytosine (which is prone to hydrolysis). Recent experiments also suggest that the original estimates of the size of an RNA molecule capable of self-replication were most probably vast underestimates.
More-modern forms of the RNA World theory propose that a simpler molecule was capable of self-replication (that other "World" then evolved over time to produce the RNA World). At this time however, the various hypotheses have incomplete evidence supporting them. Many of them can be simulated and tested in the lab, but a lack of undisturbed sedimentary rock from that early in Earth's history leaves few opportunities to test this hypothesis robustly.
"Metabolism first" models: Iron-sulfur world and others
Several models reject the idea of the self-replication of a "naked-gene" and postulate the emergence of a primitive metabolism, which could provide an environment for the later emergence of RNA replication.
One of the earliest incarnations of this idea was put forward in 1924, with Alexander Oparin's notion of primitive self-replicating vesicles, which predated the discovery of the structure of DNA. More recent variants in the 1980s and 1990s include Günter Wächtershäuser's iron-sulfur world theory and models introduced by Christian de Duve based on the chemistry of thioesters. More abstract and theoretical arguments for the plausibility of the emergence of metabolism without the presence of genes include a mathematical model introduced by Freeman Dyson in the early 1980s, and Stuart Kauffman's notion of collectively autocatalytic sets, discussed later in that decade.
However, the idea that a closed metabolic cycle, such as the reductive citric acid cycle, could form spontaneously (proposed by Günter Wächtershäuser) remains unsupported. According to Leslie Orgel, a leader in origin-of-life studies for the past several decades, there is reason to believe the assertion will remain so. In an article entitled "Self-Organizing Biochemical Cycles," Orgel (2000), summarizes his analysis of the proposal by stating, "There is at present no reason to expect that multistep cycles such as the reductive citric acid cycle will self-organize on the surface of FeS/FeS2 or some other mineral."
It is possible that another type of metabolic pathway was used at the beginning of life. For example, instead of the reductive citric acid cycle, the "open" acetyl-CoA pathway (another one of the four recognized ways of carbon dioxide fixation in nature today) would be even more compatible with the idea of self-organization on a metal sulfide surface. The key enzyme of this pathway, carbon monoxide dehydrogenase/acetyl-CoA synthase harbors mixed nickel-iron-sulfur clusters in its reaction centers and catalyzes the formation of acetyl-CoA (which may be regarded as a modern form of acetyl-thiol) in a single step.
Bubble theory
Waves breaking on the shore create a delicate foam composed of bubbles. Winds sweeping across the ocean have a tendency to drive things to shore, much like driftwood collecting on the beach. It is possible that organic molecules were concentrated on the shorelines in much the same way. Shallow coastal waters also tend to be warmer, further concentrating the molecules through evaporation. While bubbles comprised of mostly water burst quickly, oily bubbles happen to be much more stable, lending more time to the particular bubble to perform these crucial experiments.
The phospholipid is a good example of an oily compound believed to have been prevalent in the prebiotic seas. Because phospholipids contain a hydrophilic (water-soluble) head on one end, and a hydrophobic (water-repelling) tail on the other, they have the tendency to spontaneously form lipid membranes in water. A lipid monolayer bubble can only contain oil, and is therefore not conducive to harboring water-soluble organic molecules. On the other hand, a lipid bilayer bubble can contain water, and was a likely precursor to the modern cell membrane. If a protein came along that increased the integrity of its parent bubble, then that bubble had an advantage, and was placed at the top of the natural selection waiting list. Primitive reproduction can be envisioned when the bubbles burst, releasing the results of the experiment into the surrounding medium. Once enough of the "right stuff" was released into the medium, the development of the first prokaryotes, eukaryotes, and multicellular organisms could be achieved. This theory is expanded upon in the book, The Cell: Evolution of the First Organism, by Joseph Panno.
Similarly, bubbles formed entirely out of protein-like molecules, called microspheres, will form spontaneously under the right conditions. But they are not a likely precursor to the modern cell membrane, as cell membranes are composed primarily of lipid compounds rather than amino-acid compounds.
Hybrid models
A growing realization of the inadequacy of either pure "genes-first" or "metabolism-first" models is leading the trend towards models that incorporate aspects of each.
Other models
Autocatalysis
British ethologist Richard Dawkins wrote about autocatalysis as a potential explanation for the origin of life in his 2004 book, The Ancestor's Tale. Autocatalysts are substances that catalyze the production of themselves and therefore have the property of being a simple molecular replicator. In his book, Dawkins cites experiments performed by Julius Rebek and his colleagues at the Scripps Research Institute in California in which they combined amino adenosine and pentafluorophenyl ester with the autocatalyst amino adenosine triacid ester (AATE). One system from the experiment contained variants of AATE, which catalyzed the synthesis of themselves. This experiment demonstrated the possibility that autocatalysts could exhibit competition within a population of entities with heredity, which could be interpreted as a rudimentary form of natural selection.
Clay theory
A hypothesis for the origin of life based on clay was advanced by Dr A. Graham Cairns-Smith of the University of Glasgow in 1985, and adopted as a plausible illustration by just a handful of other scientists (including Richard Dawkins). Clay theory postulates that complex organic molecules arose gradually on a preexisting, non-organic replication platform—silicate crystals in solution. Complexity in companion molecules developed as a function of selection pressures on types of clay crystal is then exapted to serve the replication of organic molecules independently of their silicate "launch stage." It is, truly, "life from a rock."
Cairns-Smith is a staunch critic of other models of chemical evolution. However, he admits, that like many models of the origin of life, his own also has its shortcomings (Horgan 1991).
Peggy Rigou of the National Institute of Agronomic Research (INRA), in Jouy-en-Josas, France, reports in the February 11, 2006, edition of Science News that prions are capable of binding to clay particles and migrate off the particles when the clay becomes negatively charged. While no reference is made in the report to implications for origin-of-life theories, this research may suggest prions as a likely pathway to early reproducing molecules.
"Deep-hot biosphere" model of Gold
The discovery of nanobes (filamental structures smaller than bacteria containing DNA) in deep rocks, led to a controversial theory put forward by Thomas Gold in the 1990s that life first developed not on the surface of the Earth, but several kilometers below the surface. It is now known that microbial life is plentiful up to five kilometers below the earth's surface in the form of archaea, which are generally considered to have originated either before or around the same time as eubacteria, most of which live on the surface, including the oceans. It is claimed that discovery of microbial life below the surface of another body in the solar system would lend significant credence to this theory. He also noted that a trickle of food from a deep, unreachable, source promotes survival because life arising in a puddle of organic material is likely to consume all of its food and become extinct.
"Primitive" extraterrestrial life
An alternative to Earthly abiogenesis is the hypothesis that primitive life may have originally formed extraterrestrially, either in space or on a nearby planet (such as Mars). (Note that exogenesis is related to, but not the same as, the notion of panspermia (the notion that the seeds of life exist already in the Universe).
Organic compounds are relatively common in space, especially in the outer solar system where volatiles are not evaporated by solar heating. Comets are encrusted by outer layers of dark material, thought to be a tar-like substance composed of complex organic material formed from simple carbon compounds after reactions initiated mostly by irradiation by ultraviolet light. It is supposed that a rain of material from comets could have brought significant quantities of such complex organic molecules to Earth.
An alternative but related hypothesis, proposed to explain the presence of life on Earth so soon after the planet had cooled down, with apparently very little time for prebiotic evolution, is that life formed first on early Mars. Due to its smaller size, Mars cooled before Earth (a difference of hundreds of millions of years), allowing prebiotic processes there while Earth was still too hot. Life was then transported to the cooled Earth when crustal material was blasted off Mars by asteroid and comet impacts. Mars continued to cool faster and eventually became hostile to the continued evolution or even existence of life (it lost its atmosphere due to low volcanism). Earth is following the same fate as Mars, but at a slower rate.
Neither hypothesis actually answers the question of how life first originated, but merely shifts it to another planet or a comet. However, the advantage of an extraterrestrial origin of primitive life is that life is not required to have evolved on each planet it occurs on, but rather in a single location, and then spread about the galaxy to other star systems via cometary and/or meteorite impact. Evidence to support the plausibility of the concept is scant, but it finds support in recent study of Martian meteorites found in Antarctica and in studies of extremophile microbes. Additional support comes from a recent discovery of a bacterial ecosystem whose energy source is radioactivity (Lin et al. 2006).
The Lipid World
There is a theory that ascribes the first self-replicating object to be lipid-like. It is known that phospolipids spontaneously form bilayers in water—the same structure as cell membranes. Furthermore, these bodies may expend (by insertion of additional phospholipids) and under excessive expansion they may undergo spontaneous splitting, which preserve the same composition of lipids in the two progenies. The main idea in this theory is that the molecular composition of the lipid bodies is the preliminary way for information storage and evolution led to the appearance of polymer entities such as RNA or DNA that may store information favorably.
ReferencesISBN links support NWE through referral fees
- Brooks, J. and G. Shaw. 1973. Origins and Development of Living Systems. Academic Press. ISBN 0121357406
- De Duve, C., 1996. Vital Dust: The Origin and Evolution of Life on Earth. Basic Books. ISBN 0465090451
- Hazen, R. M. 2005. Genesis: The Scientific Quest for Life's Origins. Joseph Henry Press. ISBN 0309094321
- Horgan, J. 1991. In the beginning. Scientific American 264: 100–109.
- Huber, C. and G. Wächterhäuser. 1998. Peptides by activation of amino acids with CO on (Ni,Fe)S surfaces: Implications for the origin of life. Science 281: 670–672.
- Lee, S. H. 1981. Explaining Unification Thought. New York: Unification Thought Institute. ISBN 0960648003
- Lin, L-H., P.-L. Wang, D. Rumble, J. Lippmann-Pipke, E. Boice, L. M. Pratt, B. Sherwood Lollar, E. L. Brodie, T. C. Hazen, G. L. Andersen, T. Z. DeSantis, D. P. Moser, D. Kershaw, and T. C. Onstott. 2006. Long-Term sustainability of a high-energy, low-diversity crustal biome. Science 314: 479-482.
- Martin, W. and M. J. Russell. 2002. On the origins of cells: a hypothesis for the evolutionary transitions from abiotic geochemistry to chemoautotrophic prokaryotes, and from prokaryotes to nucleated cells. Philosophical Transactions of the Royal Society: Biological sciences 358: 59-85.
- Maynard Smith, J. and E. Szathmary. 2000. The Origins of Life: From the Birth of Life to the Origin of Language. Oxford Paperbacks. ISBN 019286209X
- Orgel, L. E. 2000. Self-organizing biochemical cycles. PNAS 97(23): 12503-12507. Retrieved February 25, 2007.
- Schopf, J. W., et al. 2002. Laser-Raman imagery of Earth's earliest fossils. Nature 416: 73-76.
- Towle, A. 1989. Modern Biology. Austin, TX: Holt, Rinehart and Winston. ISBN 0030139198
- Wells, J. 2000. Icons of Evolution: Science or Myth? Why Much of What We Teach About Evolution is Wrong. Washington, DC: Regnery Publishing. ISBN 0895262762
General subfields within Biology |
---|
Anatomy | Biochemistry | | Botany | Cell biology | Ecology | Developmental biology | Ethnobotany | Evolutionary biology | Genetics | Ichthyology | Limnology | Medicine | Marine biology | Human biology | Microbiology | Molecular biology | Origin of life | Paleobotany | Paleoclimatology | Paleontology | Parasitology | Pathology | Physiology | Taxonomy | Zoology |
Credits
New World Encyclopedia writers and editors rewrote and completed the Wikipedia article in accordance with New World Encyclopedia standards. This article abides by terms of the Creative Commons CC-by-sa 3.0 License (CC-by-sa), which may be used and disseminated with proper attribution. Credit is due under the terms of this license that can reference both the New World Encyclopedia contributors and the selfless volunteer contributors of the Wikimedia Foundation. To cite this article click here for a list of acceptable citing formats.The history of earlier contributions by wikipedians is accessible to researchers here:
The history of this article since it was imported to New World Encyclopedia:
Note: Some restrictions may apply to use of individual images which are separately licensed.